Article Information
- Digital Object Identifier (DOI): 10.47982/cgc.9. 524
- Published by Challenging Glass, on behalf of the author(s), at Stichting OpenAccess.
- Published as part of the peer-reviewed Challenging Glass Conference Proceedings, Volume 9, June 2024, 10.47982/cgc.9
- Editors: Christian Louter, Freek Bos & Jan Belis
- This work is licensed under a Creative Commons Attribution 4.0 International (CC BY 4.0) license.
- Copyright © 2024 with the author(s)
Authors:
- Giulio Cavana - Politecnico di Torino
- Brandon Mok - Loughborough University
- Matyas Gutai - Loughborough University
- Abolfazl Ganji Kheybari - Transsolar Energietechnik GMBH
Abstract
The existing building stock possesses a proportionally high impact on greenhouse gas emissions due to its low operational energy performance; retrofitting is a prominent strategy in many countries to help solve this, and as such is considered important to achieve a carbon neutral society. Among the energyretrofitting measures (ERMs), those impacting the performance of building envelopes are of primary relevance, especially ones that possess high Window-to-Wall Ratios (WWR) over 50%; these are predominantly found in office buildings built after 1960s. Here, the two dominant ERM strategies are to replace low performing elements, or to add secondary layers to the existing glass. This second strategy in particular is shown to present several benefits, however these are seldom considered and verified within a wider Life-Cycle Analysis (LCA).
To this effect, this paper aims to quantify the savings achieved through the ERM of secondary layer addition to existing glazed facades, for a high WWR office building in cooling and heating dominated climate locations (Dubai, London, and New York). This is then evaluated against an LCA for each glazing ERM chosen, to assess how each retrofit affects the embodied carbon produced. Two base scenarios in each city have been simulated in TRNSYS (single and double glass cases), with three standard market options for layers addition (single, double, and water-filled glass). Water-filled Glass is introduced as a potential ERM for the first time, that utilizes awater layer to improve performance. The aim of this paper is to evaluate the holistic efficiency of different ERMs within the transparent building envelope.
1.Introduction
The built environment is reported to be responsible for around 38-40% of global greenhouse gas emissions (around 34.9 GtCO2), and 40% of final energy consumption (128 EJ) (UN, 2020). This latter value is mostly accredited to space heating and cooling (56%) (Ariosto et al., 2019). With the push towards sustainability in construction and building sectors, there is a particular focus on the retrofit of the current building stock. In the EU, it is estimated that 85% of current building stock was constructed before 2001, and that 85-95% will still exist in 2050 (European Commission, 2020). This, paired with the fact that most of these buildings are not considered energy efficient when compared to today’s standards, means a significant number of buildings must be improved, to reduce their contribution to global emissions. At current rates, around 0.2% of buildings across the EU observe energy performance improvements of at least 60%; it is suggested that this retrofit rate must be at least doubled by 2030 (European Commission, 2020). Legislation such as Article 9 of Directive 2010/31/EU (EPBD) in the EU and Local Law 97 (LL97) in the US are encouraging this push towards more stringent building standards, by introducing carbon taxation.
A typical technique to achieve such improvements are energy retrofit measures (ERMs). This refers to incorporating efficacious practices to improve the energy and emission levels of the entire structure, whilst not compromising on the indoor climate. Within ERMs, the building envelope is crucial to consider. Depending on climate, it can be responsible for 50-60% of heat exchange and 26% of total building loads in cooling dominated climates, and 20-30% of total energy consumption (Gupta & Deb, 2023; Sarihi et al., 2021). Within the envelope, glass has a primary influence on the energy consumption in buildings, being responsible for around 20-40% of energy loss in buildings, due to high U-value and low insulative performance (Bulow-Hube, 2001).
With regards to literature surrounding ERMs, several studies (Afshari et al., 2014; Ascione et al., 2017; Aurora & Hasibuan, 2020; He & Ng, 2017; Luddeni et al., 2018; Sarihi et al., 2021; Valdiserri & Biserni, 2016) all discuss the importance of considering such a multi-faceted retrofit solution, as overall effectiveness is often limited by other envelope limitations for both cooling and heating dominated climates. This is perhaps the rationale behind retrofitting projects such as Multifunctional Energy Efficient Façade Systems (MEEFS), which due to their modular and adaptive nature can be attached to existing facades, reported to produce a 40-60% energy consumption reduction (Sarihi et al., 2021). (Sarihi et al., 2021) proposes that in cooling dominated climates, ERMs that improve the window and shading properties are highly effective (e.g., SHGC, solar shading, low-e coatings), whereas in heating dominated the concern is more around thermal envelope properties and WWR.
For glazing retrofits in particular, these are also shown to be highly effectual; in the UK, an averagereduction of 7.4% in energy use intensity may be achieved through window replacement, and 11.4% for façade replacement (UKGBC, 2024). Here, low-e is observed to be an effective solution, in the form of a coating (Edeisy & Cecere, 2017; Moghaddam et al., 2021; Somasundaram et al., 2020), and when added as a secondary glazing (Bulut et al., 2022; Smith et al., 2012). Triple glazing is also suggested to provide merits by (Hart et al., 2019; Memon, 2014) who indicate that it can offer energy demandsavings of 7-16% depending on climate. Vacuum glazing as a retrofit is assessed by (Semprini et al., 2016), reporting a 32% reduction in annual primary energy demand when single glazing is replaced. A combination of the two technologies (double and triple layer vacuum glazing) are also suggested to be effective by (Memon & Eames, 2012), however adding the third pane of glazing within a vacuum scenario provides minimal extra savings, due to the thermal transmittance being quite similar.
Whilst these studies demonstrate the operational energy advantages of glazing ERMs, they do not quantify the resultant effect on the overall LCA, nor provide information for emerging glazing technologies. This may affect the overall carbon viability of the glazing, which across the working lifespan may induce a worse scenario than what was meant to be replaced. This is also suggested by(UKGBC, 2024), who denote that the upfront embodied carbon from the replacement of windows can represent 50-100% of whole-life operational savings.
To resolve this, this paper simultaneously investigates the LCA and operational energy impact of secondary glazing additions on an office space, across both cooling and heating dominated climates.Secondary glazing is not covered in the report discussed above, and as such this paper will contribute to this gap in research. Further novelty is created from the investigation of novel hybrid options such as Water-filled glass (WFG) for the first time, measured against other standard glazing options. WFGmakes use of a water infill layer to capture, absorb, and reuse incoming radiation that would normally be lost, in order to reduce cooling load and ensure thermal comfort of occupants.
2. Methodology
To best express the methodology used within this study, it has been divided into four subsections, namely considerations for building simulation model, mechanical scenario, window options, and LCA. These will be explored below.
2.1. Considerations for Building Simulation Model
In this study, an office cubicle prototype has been modelled, akin to what would be found in a typical high-rise building. To provide an analysis that is representative of most of the building, an intermediate space not connected to external side walls, roof, or ground has been selected. The equatorial orientedSouth-facing wall was the only wall selected to provide an external interaction with the ambient environment, with the rest considered adiabatic; this is assuming that the adjacent office spaces havethe same air-conditioned thermal setpoints. The glazed face on the external wall was modelled as9.2m2 (3.3m x 2.8m) with a Window-to-Wall (WWR) ratio of 88%, and the floor and ceiling area considered was 17.5m2. This office space can be observed in Figure 1 below.
The construction type of the internal partition walls was represented by a typical office scenario; two layers of gypsum board with insulation, creating an average U value of 0.317 W/m2K, with a thickness of 0.126m. The external wall was simulated to have a U value of 0.191 W/m2K, made from a combination of gypsum, air, insulation, and concrete (around the glazing space), with a thickness of 0.413m. The ceiling has a U value of 0.581 W/m2K, and the floor the same.
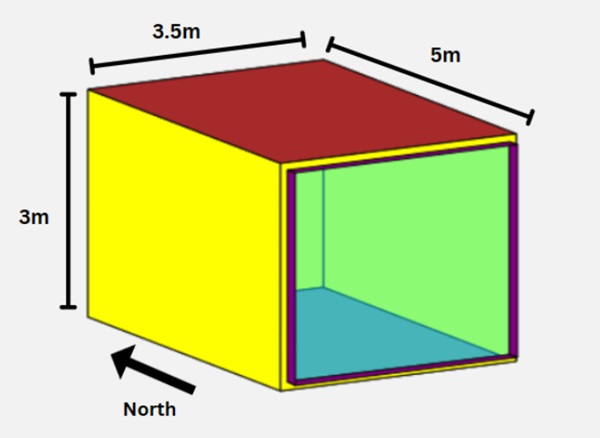
2.2. Considerations for Mechanical Scenario
The mechanical scenario that was simulated is as follows. The geopositioned air nodes within the zonewere chosen at 2m interval distance along the y axis, at 0.8m reference plane height (desk height). This was done to best stratify the sub-areas of the space that experience different temperatures. The overall office space was programmed to start at 20oC and 50% humidity, the latter remaining constant via the capacitance model feature in TRNSYS. Whilst this may underreport de/humidification load, due to not including moisture buffering, these were deemed low in comparison to heating and cooling requirements in this study (Steeman et al., 2009).
The working hours of the simulation were assumed to be 0800 to 1800, to simulate a normal workday, with no occupancy recorded at weekends. Infiltration rates (n=1.45 during working hours and n= 0.25 ACH otherwise) and internal heat gains were also assumed to follow this schedule, to match the manual opening of windows. Ventilation was achieved through night flushing (n=5 ACH during summertime), and internal gains were represented by persons (130 W/m2), lighting (5 W/m2, 300 lux), and electrical equipment (140 W/m2). For the heating and cooling scenario, these were considered to be ideal, with no restriction on power.
The locations chosen were Dubai, London, and New York. This was to cover both cooling and heating dominated climates, of which each has a significant number of glazed office buildings.
2.3. Considerations for Window Options
Eight different windows were simulated in this study: two base cases and six retrofit strategies. The rationale behind each of these are as follows. Firstly, for the two base cases Single Glass (SG_Base) and Double Glass (DG_Base), these were chosen to simulate the majority of the building stock needing retrofitted, of which both of these are common techniques. This will allow a direct comparison to which retrofit strategy provides the most merit.
The first retrofit scenario for each base case is the addition of a single glass layer to the existing unit, thus resulting in a Double Glass unit (SG_SG) and in a Triple Glass (DG_SG). In a similar manner, double glass has also been simulated as secondary glazing, creating SG_DG, and DG_DG. The secondary glazing is placed on the inside of the existing glazing, rather than on the outside of the transparent envelope. This poses a more economically viable option (since no external works or protection is required), it is non disruptive for occupants, respects the heritage of building envelope, has the same operational properties as if it was placed externally, and is the typical choice within the industry.
Next, an advanced glazing (WFG) has been modelled as a secondary glazing, resulting in retrofit scenarios of SG_WFG and DG_WFG. This is a novel aspect, and has not been considered in a retrofit scenario before. WFG is a technique whereby a water infill layer is utilised as a heat absorption layer to not only control and capture incoming radiation, but prevent the loss of heat from the internal environment (Gutai & Kheybari, 2021). This aims to provide energy savings via a mechanical system, either through expelling the wasted heat back into the environment, or for domestic hot water systems or heat reuse.
The glass retrofit compositions, including their spectral and thermal properties, can be seen below in Table 1. The second column provides the glazing composition, with the thickness of each layer includedin millimetres. The leftmost value represents the outside-facing pane, with the rightmost value being the inside pane. The bold numbers represent the glazing thickness, with the gas layers and any coatingsdescribed in the third column.
Table 1: Thermal and optical properties of glazing retrofit systems.
2.4. Considerations for LCA framework
For this study, the calculations follow a cradle-to-grave framework, to quantify emissions over the entire lifecycle, cradle-to-grave (Stages A1-C4).
For the Product Stage (A1-A3), the Inventory of Carbon and Energy (ICE, 2019) provided information to base the embodied carbon calculations off for the materials used for each glazing scenario. Within this phase, generic elements within the office space were not considered, since these are not part of the retrofit. As such, only the window replacement is accounted for. For each of the window ERMs, 100:0 calculation method was used, to align with the EPDs used for the glass options. For the WFG style retrofits, no EPD exists for these; the additional components such as heat exchanger were accounted for manually. For the Construction Stage (A4-A5), it assumed the same transportation distances (national) to the place of construction, to follow the guidelines presented by (RICS, 2022).
Here, the authors presume the technologies for the actual construction process are relatively similar, and as such will have a comparative negligible difference.
The embodied carbon from the Use Stage (B1-B5) was considered low for this scenario. B1, B2, B3, B5 (use, maintenance, repair, and refurbishment) would be insignificant, since the glazing space is being retrofitted in this study already via means of a secondary glazing. For B4 (replacement), this has been assumed to not contribute to the overall carbon, since the lifespan of the retrofitted glazing coincides with the Reference Study Period (RSP) chosen (50 years).
C1-C4 were also represented in this study, with typical transportation distances to waste facilities calculated for each location, again following guidelines from (RICS, 2022).
3. Results
This section will compare the overall embodied energy with the operational performance of each glazing ERM. The total annual energy intensity outputted by the TRNSYS analysis was converted into annual energy consumption by applying Coefficient of Performances (COP) for heating (4.2), cooling (4), and electricity (1). Next, this was transformed into primary energy, through site-to-source energy conversion factors; these were assumed to be 3.31 throughout the study (EN 15603). Finally, using specific carbon coefficients for each location, this provided the overall operational emissions created through each retrofit. This can be observed below in Table 2.
Table 2: The annual energy intensity, consumption, and CO2 emissions for glazing retrofits in three cities.
The operational emissions of each glass retrofit can also be seen below, provided to allow for easier comparisons.
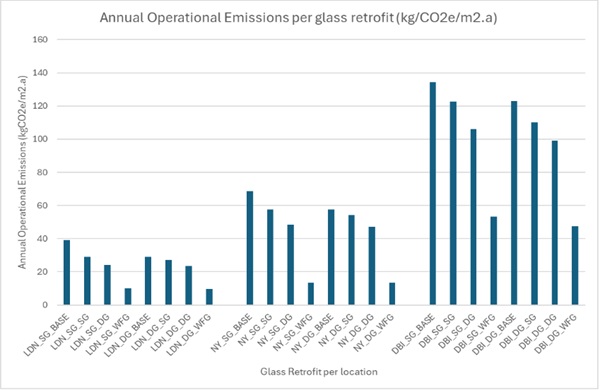
This can then be compared against the embodied carbon created through each retrofit, as shown below in Figure 3. Note that the base cases for each scenario are represented by zero embodied carbon; this simply means that comparatively, no additional carbon is presented by this technique, as no retrofit is taking place here. Toughened glass has been modelled for each technique, to provide a standard scenario for commercial buildings with larger WWR.
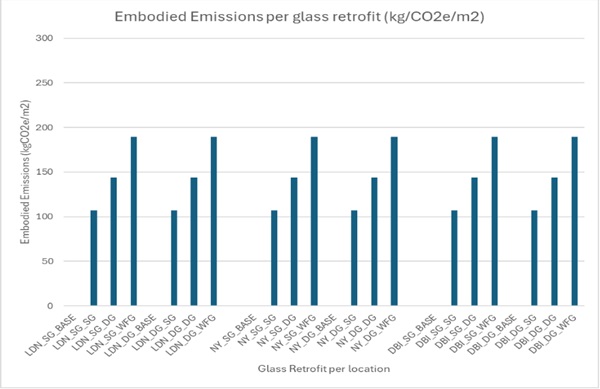
Finally, the carbon payback periods have also been calculated. It is worth noting that these calculations assume the weather data, carbon coefficients, or the COPs to not change during the study period considered. However, this was deemed insignificant since most payback periods are under ten years.In Figure 4 below, the operational carbon savings are weighted against the increase in embodied carbon, to assess the viability of the ERM. DG_SG retrofit for London has been omitted from this graph, since the technique actually incurs an additional energy consumption rather than a saving. This will be discussed below.
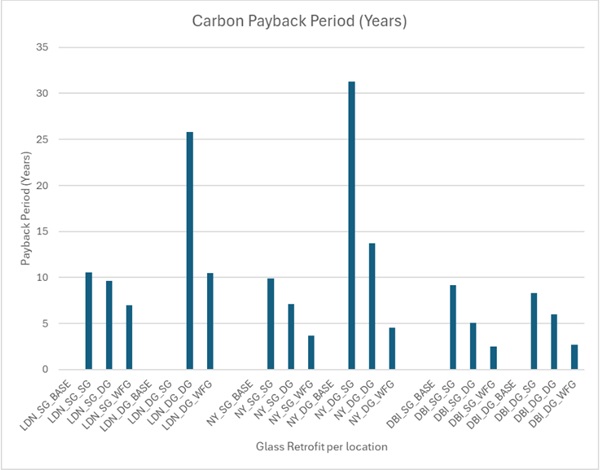
4. Discussion of results
This study analysed the carbon viability of different retrofit ERMs for glazing, through TRNSYS simulations on an office space. These results are significant, since office spaces are in need of dire retrofit across the globe; at least 16% of EU countries’ worst performing non-residential buildings must be retrofitted by 2030 (EU, 2023). These results aim to elucidate the potential benefits of ERM window retrofitting, and which techniques likely hold most merit.
For all three climates studied, the window retrofit with both the lowest payback period and carbon emissions is WFG. Depending on location, for SG_Base this comparatively saves between 60-80% carbon emissions with carbon payback periods of 2.5-7 years. Similarly, for DG_Base retrofits, WFG again presents the most optimum solution, with 65-81% emission savings with 2.7-10.5 years of carbon payback. With regards to its embodied carbon however, WFG possesses the highest out of any secondary glazing addition. This is due to its thick glazing, the additional requirements of spacer and provision of low-e coatings. Nonetheless, as indicated above, this is quickly recovered in the payback period and operational savings. In addition, it is worth noting that WFG and similar technologies can store and harvest energy from solar irradiation. This would help reduce the overall energy consumption of the indoor environment, by either using periods of discomfort to transfer energy to solve other discomfort (e.g., redirecting heat captured on South façade to assist cooling, and also to assist heating on North façade), or to supplement domestic hot water demand. This has not been included within this paper, purely to provide a better comparison and understanding of technique viability.
The second-best retrofit technique for each climate, in terms of operational savings and payback period, is adding double glazing. This provides admirable savings across all climates (21-40%). With regards to which technique has the highest operational emissions, this is found when adding a single glazing secondary layer, producing annual carbon emission savings of only 9-18% depending on climate and base case. For double glass with a single glazing retrofit, this actually incurs an additional energy usage in London, rather than a saving. As such, the payback period becomes somewhat irrelevant, and it is not worth using from a carbon perspective.
Whilst the carbon savings are important, these must be weighed against the financial cost required to achieve these. It is estimated that a typical window replacement costs on average £110/ m2 GIA, and façade replacement £640/m2 GIFA (UKGBC, 2024). The cost payback period for this is dependent on the operational energy demand for the office space; on average, the annual bill equates to £37/sqm for London (Lambert Smith Hampton, 2023).
According to the above literature, a window replacement can provide a 7.4% average EUI reduction. However, even with this, to offset the window replacement, a 41-year payback period is required. Since this is outside the typical lifespan of a glazing (35 years), this points towards the need for a more efficient retrofit, that is several times more efficient. With regards to the façade replacement, provides an even longer cost payback; with an 11.4% EUI reduction, this payback would be higher than the building life itself (155 years).
To this effect, the present study proposes that WFG is a potential solution to this, as with up to 81% annual EUI reductions (depending on base case and climate), it can help promote a viable carbon and cost payback. Considering the studies above and using London as an example, £27/ m2 GIA can be saved annually when retrofitting either single or double glass with WFG, resulting in a viable cost payback period of just over three years. In addition, due to the technique being added as secondary glazing, the cost would be reduced even further, since the need for scaffolding and complex external works is avoided.
4.1. Error Analysis
In order to address any potential errors the software can make, validation for the TRNSYS model was undertaken before conducting the simulation. For the standard window options, data for these is readily available, and as such already exists within the LBNL Window library. The importing processfrom LBNL Window to TRNSYS will be explained later in the text. For WFG, this must be validated independently (due to its novelty and uniqueness of interlayer design), as discussed below.
To provide the optical characteristics of WFG, Beer Lambert’s Law was used to calculate the absorption and transmission of the water layer. Here, the thickness of the water layer directly influences these variables. The thermal collection properties (e.g., molecular weight, conductivity, viscosity, specific heat coefficient), and light transmittance potential for different water thicknesses were quantified via previous research by the authors, assuming ASTM E-490 Standard Spectra.
To produce such a window for TRNSYS simulation, LBNL Window (v7.6) was used to model thesethermal and optical behaviours. Here, the former (thermal conductivity of water) was introduced into the library as a new gas, whilst the latter was first defined in Optics 6, and then implemented within Window as a shading material. To import these behaviours into TRNSYS, they were converted into BSDF (Bidirectional Scattering Distribution Function) datasets, using trnBSDF tool. This combines such datasets with other gap and layer information, in an overall form of a Klems matrix (145x145). This modelling approach is considered one of the most accurate for complex fenestration systems, enabling the precise calculation of radiative heat flux, absorbed solar radiation, and temperature for individual layers and gaps of the glazing system.
Field tests and monitoring were also conducted previously, that could validate the simulation for WFG. Firstly, a study using the Water House 2.0 Pavilion in Taichung, Taiwan, confirmed the thermal effect of water flow in an optically transparent setting. During the monitoring period, the building's temperature, both indoors and on surfaces, notably decreased when the pump was operational compared to when it was turned off. This cooling effect was solely attributed to the water flow, as no other cooling system was in operation, and ambient temperature and solar gain remained consistent in both scenarios.
Secondly, a small prototype of WFG was tested with a Klipp and Zonen SP Lite pyranometer model.Measurements were conducted outdoors to assess natural solar gain, employing both horizontal and vertical settings. The glass was measured both with and without water infill. The observed change in Solar Heat Gain Coefficient (SHGC) after water infill closely correlated with the simulation. Additionally, the changes in the visible spectrum were confirmed using an LP Standard Pro spectrometer, which indicated no difference in absorption within the visible spectrum.
5. Conclusions
This study seeks to elucidate the potential merit of retrofitting the current building stock, in particularwindows to increase operational performance. This is completed through using simulation-basedsoftware TRNSYS, to model an office environment and assess the operational energy consumption induced by various window retrofits, across both cooling and dominated climate types. The study concludes that there is significant merit to inserting additional panes of glazing. If the office space has single glazing, then the most effective strategy is shown to be adding WFG as a secondary glazing (60-80% carbon saving); for pre-existing double glazing, a WFG secondary layer can provide between 65-81% savings. The lowest carbon payback periods are also experienced by WFG retrofits, between 2.5-10.5 years.
References
Afshari, A., Nikolopoulou, C., & Martin, M. (2014). Life-Cycle Analysis of Building Retrofits at the Urban Scale—A Case Study in United Arab Emirates. Sustainability, 6(1), 453–473. https://doi.org/10.3390/su6010453
Ariosto, T., Memari, A. M., & Solnosky, R. L. (2019). Development of designer aids for energy efficient residential window retrofit solutions. Sustainable Energy Technologies and Assessments, 33, 1–13. https://doi.org/10.1016/j.seta.2019.02.007
Ascione, F., Bianco, N., Masi, R. F. De, Perone, T., Ruggiero, S., Strangio, P., & Vanoli, G. P. (2017). Light and Heavy Energy Refurbishments of Mediterranean Offices. Part II: Cost-optimal Energy Renovation of an Institutional Building. Procedia Engineering, 180, 1518–1530. https://doi.org/10.1016/j.proeng.2017.04.314
Aurora, D. M., & Hasibuan, H. S. (2020). Retrofit Plans on Building Envelopes as Energy Efficiency Efforts: A Green Building Concept. IOP Conference Series: Earth and Environmental Science, 448(1), 012058. https://doi.org/10.1088/1755-1315/448/1/012058
Bulow-Hube, H. (2001). Energy-efficient window systems-effects on energy use and daylight in buildings.
Bulut, M. B., Wilkinson, S., Khan, A., Jin, X.-H., & Lee, C. L. (2022). Thermal performance of retrofitted secondary glazed windows in residential buildings – two cases from Australia. Smart and Sustainable Built Environment, 11(4), 1182–1192. https://doi.org/10.1108/SASBE-03-2021-0050
Edeisy, M., & Cecere, C. (2017). Envelope Retrofit in Hot Arid Climates. Procedia Environmental Sciences, 38, 264–273. https://doi.org/10.1016/j.proenv.2017.03.075
EU. (2023). ‘Fit for 55’: Council and Parliament reach deal on proposal to revise energy performance of buildings directive.
European Commission. (2020). A Renovation Wave for Europe.
Gupta, V., & Deb, C. (2023). Envelope design for low-energy buildings in the tropics: A review. Renewable and Sustainable Energy Reviews, 186, 113650. https://doi.org/10.1016/j.rser.2023.113650
Gutai, M., & Kheybari, A. G. (2021). Energy consumption of hybrid smart water-filled glass (SWFG) building envelope. Energy and Buildings, 230, 110508. https://doi.org/10.1016/j.enbuild.2020.110508
Hart, R., Selkowitz, S., & Curcija, C. (2019). Thermal performance and potential annual energy impact of retrofit thin-glass triple-pane glazing in US residential buildings. Building Simulation, 12(1), 79–86. https://doi.org/10.1007/s12273-018-0491-3
He, Q., & Ng, T. (2017). Energy-efficient window retrofit for existing high-rise residential buildings with the consideration of mutual shading. Association of Researchers in Construction Management.
ICE. (2019). Embodied Carbon - The ICE Database.
Lambert Smith Hampton. (2023). Key Findings 2023. https://www.lsh.co.uk/total-office-cost-survey
Luddeni, G., Krarti, M., Pernigotto, G., & Gasparella, A. (2018). An analysis methodology for large-scale deep energy retrofits of existing building stocks: Case study of the Italian office building. Sustainable Cities and Society, 41, 296–311. https://doi.org/10.1016/j.scs.2018.05.038
Memon, S. (2014). Analysing the potential of retrofitting ultra-low heat loss triple vacuum glazed windows to an existing UK solid wall dwelling. International Journal of Renewable Energy Development, 3(3), 161–174. https://doi.org/10.14710/ijred.3.3.161-174
Memon, S., & Eames, P. (2012). HEAT LOAD AND SOLAR GAIN PREDICTION FOR SOLID WALL DWELLINGS RETROFITTED WITH TRIPLE VACUUM GLAZING FOR SELECTED WINDOW TO WALL AREA RATIOS. World Renewable Energy Forum , 4636–4643.
Moghaddam, S. A., Mattsson, M., Ameen, A., Akander, J., Gameiro Da Silva, M., & Simões, N. (2021). Low-Emissivity Window Films as an Energy Retrofit Option for a Historical Stone Building in Cold Climate. Energies, 14(22), 7584. https://doi.org/10.3390/en14227584
RICS. (2022). Whole Life Carbon Assessment for the Built Environment, RICS Professional Standard, 2nd edition.
Sarihi, S., Mehdizadeh Saradj, F., & Faizi, M. (2021). A Critical Review of Façade Retrofit Measures for Minimizing Heating and Cooling Demand in Existing Buildings. Sustainable Cities and Society, 64, 102525. https://doi.org/10.1016/j.scs.2020.102525
Semprini, G., Marinosci, C., Ferrante, A., Predari, G., Mochi, G., Garai, M., & Gulli, R. (2016). Energy management in public institutional and educational buildings: The case of the school of engineering and architecture in Bologna. Energy and Buildings, 126, 365–374. https://doi.org/10.1016/j.enbuild.2016.05.009
Smith, N., Isaacs, N., Burgess, J., & Cox-Smith, I. (2012). Thermal performance of secondary glazing as a retrofit alternative for single-glazed windows. Energy and Buildings, 54, 47–51. https://doi.org/10.1016/j.enbuild.2012.07.038
Somasundaram, S., Chong, A., Wei, Z., & Thangavelu, S. R. (2020). Energy saving potential of low-e coating based retrofit double glazing for tropical climate. Energy and Buildings, 206, 109570. https://doi.org/10.1016/j.enbuild.2019.109570
Steeman, M., Goethals, K., Laverge, J., Janssens, A., & Paepe, M. (2009). ON MODELLING MOISTURE BUFFERING WHEN EVALUATING HUMIDITY CONTROLLED HVAC SYSTEMS. Eleventh International IBPSA Conference.
UKGBC. (2024). Building the case for Net Zero: Retrofitting Office Buildings.
UN. (2020). 2020 GLOBAL STATUS REPORT FOR BUILDINGS AND CONSTRUCTION FOR BUILDINGS AND CONSTRUCTION.
Valdiserri, P., & Biserni, C. (2016). Energy performance of an existing office building in the northern part of Italy: Retrofitting actions and economic assessment. Sustainable Cities and Society, 27, 65–72. https://doi.org/10.1016/j.scs.2016.07.009
Comments
That's a really interesting and important area of research. Looking at the full carbon footprint of building retrofits, especially with innovative materials like water-filled glass, is key to making sustainable choices for our buildings.
-Moving Company Cincinnati
In London, retrofitting double glass with single glazing actually results in higher energy consumption rather than savings. As a result, the payback time loses some of its significance and is not worthwhile from a carbon standpoint.