This paper was first presented at GPD 2019 by Marco Zaccaria and Xavier Gillon from AGC Glass Europe – Technovation Center, Gosselies, Belgium.
AGC recently developed a special glass composition (commercial name Falcon glassTM) that can be produced by a standard float line and on which chemical tempering is very effective.
The convenient price due to standard float production alongside with the excellent mechanical properties arising from chemical tempering make Falcon glassTM suitable for unprecedented thin glass applications in architecture. In order to explore the potential of Falcon glassTM destructive tests were performed to evaluate the failure stress of glass under different tempering conditions and when subjected to ageing.
Reference soda-lime-silica glass was also tested. It was shown that Falcon has excellent mechanical properties after chemical tempering and that its stress at failure does not decrease when subjected to artificial ageing, in contrast to what happens for soda-lime-silica glass. Different chemical tempering settings were also explored, showing that longer treatments do not impact the stress at failure, but they allow to build a larger case depth, which improves further the ageing resistance.
1. Factors limiting the use of thin glass in architecture
In the last decade thin chemically tempered (CT) aluminosilicate glass (ASG) has been widely used as display in the booming market of smartphones and tablets. Aluminosilicate glass is commonly produced by overflow fusion or down-draw process, which allow to produce glass as thin as 0.1 mm. Chemical tempering process consists of immersing the glass into a melted KNO3 salt bath (at about 400°C - 460°C) for a given amount of time (commonly between 1 – 24 hrs) [1]. The conditions during chemical tempering favour K+ in the bath to replace smaller Na+ in the glass structure. The shrinkage of potassium ions to occupy the place of sodium ions generates a compression on the glass surface that can be as high as 1000 MPa [2].
The high surface compression alongside with the slenderness of the panel gained the interest of engineers, architects and researchers to exploit the potential of this material in architecture. Overend et al. [3] studied the strength and the post-fracture performance of a triple laminated assembly comprising thin CT-ASG as outer face and conventional soda-lime-silica glass (SLSG) in the core.
Lambert and O’Callaghan [4] identified three possible field of applications for thin glass: laminated plies where one or more layers are made of high-strength thin glass (for anti-explosion for example); cold bent panels; tensile membrane structures. The slenderness of the glass suggested to study movable façade elements, such as canopy [5] or adaptive façade panels [6]. Experiments to exploit the mechanical strength of the glass whilst limiting its deformation were made by Akilo [7] who combined in a composite panel thin CT-ASG with a 3D printed plastic core and by Guidi [8] who added the cold bending feature to the work of Akilo.
However, there are two main reasons why thin glass has not yet boomed in the architectural world, namely:
- ASG is too expensive;
- there is lack of confidence in the long-term strength of CT glass.
ASG is too expensive because the raw materials need much higher temperature for melting than SLSG, which implies that the oven requires higher quality refractories (i.e. more expensive) and its operational life is shorter than that of a standard float. Furthermore, the production yield is much lower compared to a standard float line.
SLSG does not benefit from ion exchange as much as ASG does. Datsiou and Overend [9] tested artificially aged CT SLSG, finding a significant decrease in strength, due to artificially generated flaws being larger than the case depth (25-30 µm only [10]). However, the case depth in CT ASG can be as high as 100 µm [11], suggesting that a glass with such a case depth would be much more reliable against ageing, but was not extensively tested to date.
Therefore the question: is there a thin glass cheaper than ASG but capable to build-up a residual stress with a case depth larger than that of SLSG?
2. Falcon glassTM
Falcon glassTM (FG) is produced by AGC on a standard float line. It has a specific chemical composition which is specifically tailored to maximize its mechanical performances after chemical tempering, while maintaining melting temperatures compatible with standard float. It is produced in thickness of 0.5, 0.7, 1, 1.1, 1.3, 1.6, 2.0, 2.1, 3, and 4 mm.
Falcon’s potential of developing surface compression and case depth compared to ASG and SLSG is illustrated in Figure 1. It can be observed that FG outperforms entirely SLSG and partially overlaps with ASG offering an unprecedented trade-off between cost and efficiency of chemical tempering. The chemical differences between the tin and air side are such to influence the rate of ion-exchange, therefore an anti-warpage treatment is needed to balance the reactions and ensure the flatness of the panels after ion-exchange Figure 2. A summary of FG’s properties in Table 1.
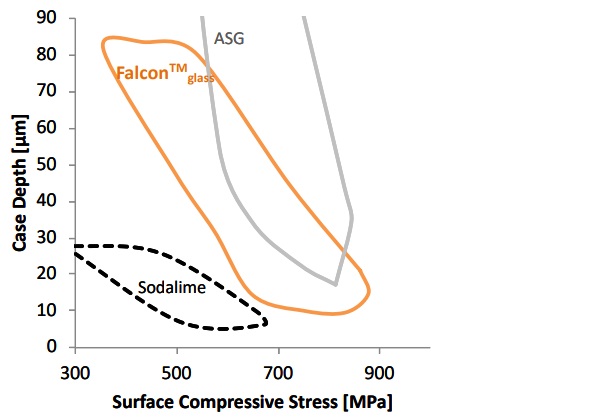

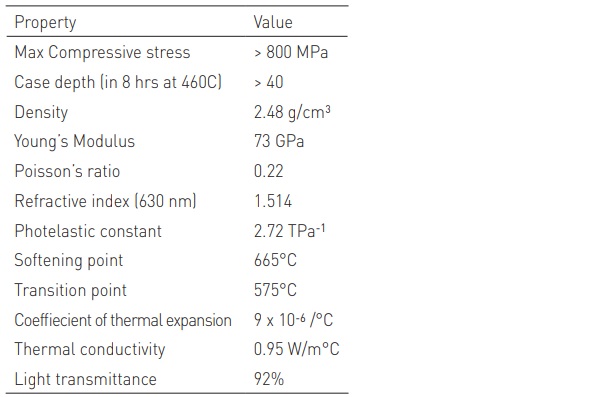
There are few challenges in processing thin glass that should be taken into account: - Cutting and grinding;
- Cutting and grinding;
- Coating;
- Lamination.
It is possible to hand-cut thin glass, but due to its thickness specific tooling and full flat and rigid surface support is needed. To ensure a good edge-quality it is preferable to use techniques capable of a good edge finish without further processing (i.e. laser-cut). Lamination and coating on thin glass have no particular restriction at a theoretical level, but the industrial equipment that is actually used for standard glass might not be capable of handling thin glass. On this subject industrial trials are on-going.
3. Mechanical testing
Recent studies highlighted the difficulty of testing destructively thin glass [12], [13], [14]. The glass is indeed so thin that it undergoes large deformation during testing: in these conditions the theory and the actual stress do not match (Figure 3). The most promising methods for testing thin glass consists of pure tensile test [14] or constant radius bending [13]. However, 2.1 mm thick glass can still be tested with the conventional coaxial double ring (CDR) or 4-point bending.
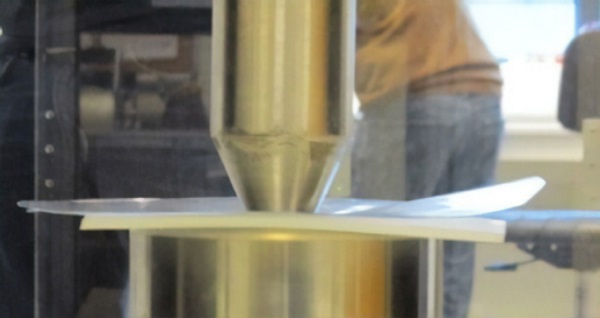
3.1. Coaxial Double Ring Test for 2.1 mm thick glass
Seven series of 100 x 100 x 2.1 mm glass were tested using a CDR. The series comprised of CT and non-CT FG and SLSG. The CT series included two different chemical tempering cycles and two series of artificially aged glass by means of sand-abrasion. The chemical tempering cycles are 460°C for 8 hours and 460°C for 24 hours.
The sand abrasion consisted of 0.90 m high fall of 750 g of sand, with 500 µm average grain size, impacting the air side of the glass at an angle of 45°. After sand abrasion the glass was kept for 24 hours in lab conditions before destructive testing. A summary of the series is shown in Table 2. Before the CDR test a transparent adhesive film was placed on the tin side of the glass to keep the glass fragments together after failure and locate so the origin of failure. Specimens were always tested with the air side in tension.
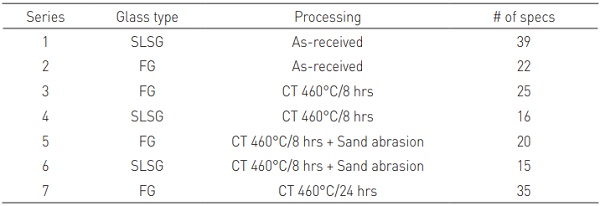
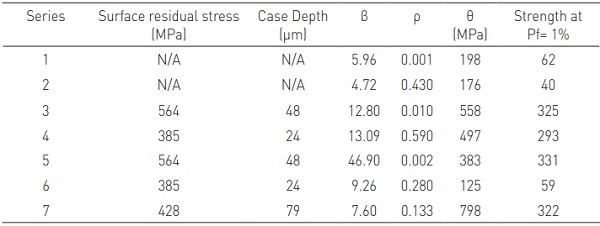
The CDR setup was installed on an Instron machine fitted with a 30 kN load cell. The loading and reaction ring measured 20 and 80 mm in diameter, respectively. The test was performed by applying a stress rate of 2 MPa/s. Load at failure was recorded and stress at failure determined according to the elastic theory, as described by Zaccaria and Overend [15], although slow crack growth was not accounted for in the current study. Two-parameter Weibull statistical analysis was performed on the failure stress data to identify the failure stress for a given probability. Namely, 1% probability of failure is reported in Table 3 along with Weibull parameters, average surface residual stress and relative case depth. The Weibull parameters are:
- β is the shape parameters, which represents the gradient of the best-fit curve, when data are log-plotted;
- ρ is an indication of the goodness of fit. Values > 0.05 indicate poor fit. (Poor fit series are in italics in Table 3);
- θ is the scale parameters and is the failure stress value which corresponds to Pf = 63.21%.
Residual stress and relative case depth were measured on the air surface of each specimen by means of a differential stress refractometer (DSR).
The fracture pattern of the broken specimens did not show potential of spontaneous breakage as per thermally tempered glass (Figure 4), therefore safety thin glass ought to be laminated glass.
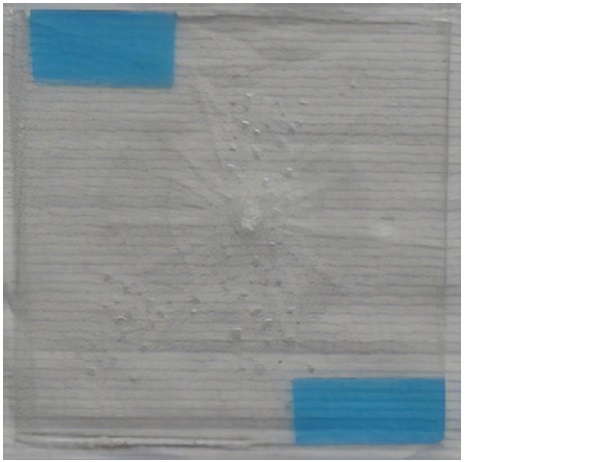
4. Discussion
Falcon glass is stronger than SLSG when tempered with the same CT cycle and it generates a larger case depth (double for 8 hours at 460°C). Considering series 3 and 4, at Pf = 1% it can be observed that the failure stress of FG is about 10% larger than that of SLSG (i.e. 325 MPa against 293 MPa, respectively, Figure 5). Although the actual gain of FG over SLSG arise from the artificially aged series, i.e. series 3 with 5 and series 4 with 6, respectively.
Falcon glass was not affected by ageing. It can be observed that the failure stress for FG at Pf = 1% FG 325 MPa and 331 for the CT only and the CT + sand abrasion series, respectively, whilst SLSG failure stress is 293 and 59 MPa, respectively (Figure 6). This means that the artificial ageing deleted the surface residual stress returning a glass which has the same performances of non-CT asreceived SLSG (series 1). This can be explained by the difference in case depth 48 and 24 µm, respectively. Smaller case depth means higher impact of the flaws on the failure stress of glass. Comparing artificially aged FG with SLSG it can be concluded that at Pf = 1% FG failure stress is 5 times higher than SLSG.
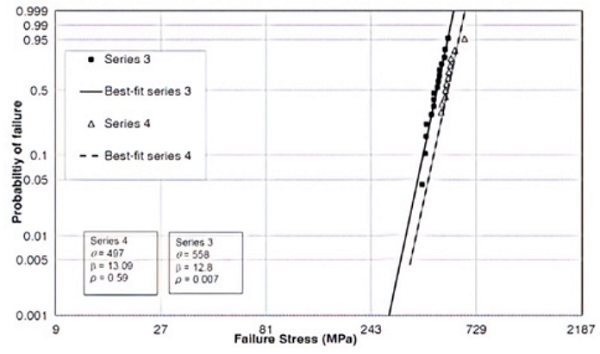
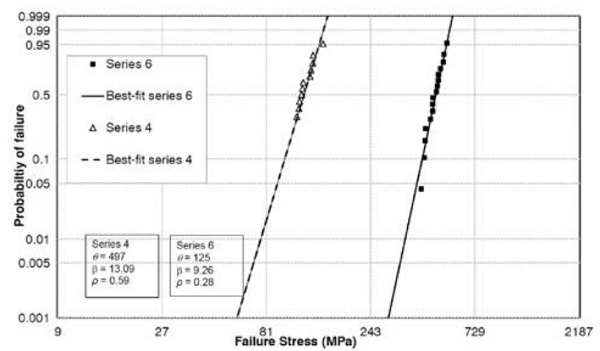
Another interesting result arises when comparing FG with different tempering cycles (Figure 7). The failure stress at Pf = 1% is almost the same (325 and 322 MPa for 8 and 24 hours, respectively). However, the case depth is much larger in the cycle lasting 24 hours, 48 vs 78 µm, respectively. This suggest that FG undergoing a 24 hours ion exchange at 460°C is stronger than FG tempered for 8 hours at 460°C despite this difference is not perceivable without ageing. In terms of surface residual stress it can be noted that FG can build-up much larger surface stresses than SLSG (564 vs 385 MPa for FG CT and SLSG CT, respectively for 8 hours at 460°C) and that longer tempering time generates a stress relaxation as the surface residual stress decreases from 564 to 428 MPa for FG tempered for 8 and 24 hours.
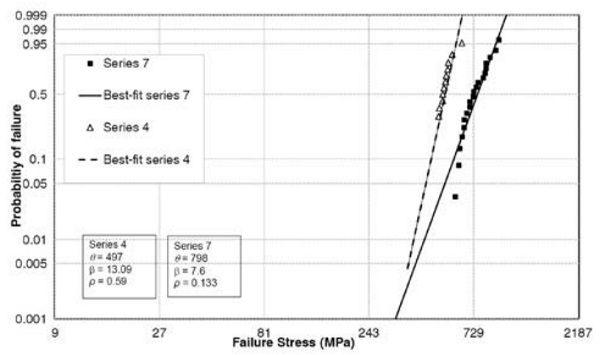
It should be noted that the sand abrasion technique used was not calibrated, in the sense that it was not studied how the artificial sand abrasion relates to a natural ageing reference time. However, it is clear that larger case depth ensures a protection against ageing. Large case depth can be achieved by float produced FG, chemically tempered for 24 hours. Furthermore, if for example FG is to be used in the core of a laminated unit, its surface would be protected by the outer plies and therefore even smaller case depth (i. e. obtained by ion exchange for 8 hours at 460°C) would be effective to ensure failure stress which would not be affected by ageing.
5. Conclusion and future work
AGC succeeded to produce Falcon glass, a float thin glass with enhanced mechanical performances after undergoing ion-exchange. Falcon glass was tested using coaxial double ring and the results were compared with standard soda-lime-silica glass. It was observed that Falcon glass is stronger than soda-lime-silica (325 vs 293 MPa, respectively at Pf = 1%). The difference in stress at failure is even larger when comparing artificially aged series (331 vs 59 MPa, respectively, i.e. 5 times higher).
This can be explained by the case depth, which in falcon glass, for the settings used in this study, is about double than that of soda-lime-silica glass when tempered with the same conditions (48 and 24 µm, respectively). When comparing Falcon glass subjected to ion exchange with different parameters (i. e. 460 °C for 8 and 24 hours, respectively) it can be observed that the failure stress at Pf = 1% does not change, although the case depth does (48 vs 79 µm, respectively). This would suggest that when processing Falcon glass to a longer ion-exchange for a given temperature, ageing resistance will increase.
The scientific interest on thin glass is constantly rising and the amount of work to be done in order to use it in real applications is still relevant. AGC is currently exploring the following fields:
- Thin glass handling and processing (i.e. cutting, grinding, coating, lamination, moving, transportation);
- Thin glass properties exploration (i.e. tensile testing, constant radius testing, cold bending, lamination with inserts, chemical tempering);
- Thin glass application in different fields, namely marine, lightweight IGUs, cold bending, and challenging applications.
Acknowledgements
The author would like to thank researchers and technicians of the Glass Melting & Refractories and Transformation department of the Technovation centre for their precious work and advices.
References
[1] R. F. Bartholomew and H. M. Garfinkel, “Chemical strengthening of glass,” Glass Science and Technology, D.R. Uhlmann and N.J. Kreidl Vol. 5 New York.
[2] A. K. Varshneya, “Chemical Strengthening of Glass: Lesson Learned and Yet To Be Learned,” International Journal of Applied Glass Science, vol. 1, no. 2, pp. 131-142, 2010.
[3] M. Overend, C. Butchart, J. O’Callaghan and M. Prassas, “Glass reinforced glass,” Glass Performance Days, 2013.
[4] H. Lambert and J. O’Callaghan, “Ultra-thin High Strength Glass Research and Potential Applications,” Glass Performance Days, pp. 95-99, 2013.
[5] J. Neugebauer, “A movable canopy,” International Conference on Building Envelope Design and Technology. Advanced Building Skins, pp. 318-325, 2015.
[6] R. Ribeiro Silveira, C. Louter and T. Klein, “Flexible transparency - A study on Adaptive THin Glass Facade Panels,” Challenging Glass Conference, vol. 6, pp. 135-148, 2018.
[7] M. Akilo, “Design and analysis of a composite panel with ultra-thin glass faces and a 3D-printed polymeric core,” Msc thesis, University of Bologna, 2018.
[8] M. Guidi, “Thin Glass Cold Bent Sandwich Panel,” MSc Thesis, TU Delft, 2019.
[9] K. Datsiou and M. Overend, “The strength of aged glass,” Glass Structures and Engineering, 2017.
[10] A. K. Varshneya, “The physics of chemical strengthening of glass: Room for a new view,” Journal of Non-Crystalline Solids, vol. 356, pp. 2289-2294, 2010.
[11] A. J. Burggraaf and J. Cornelissen, “The strengthening of glass by ion-exchange. Part I. Stress formation by ion diffusion in alkali aluminosilkicate glass,” Physics and Chemistry of Glasses, vol. 5, no. 5, pp. 123-129, 1964.
[12] I. Maniatis, G. Nehring and G. Siebert, “Studies on determining the bending strength of thin glass,” Structures and Buildings, pp. 393-402, 2014.
[13] J. Neugebauer, “Determining of Bending Tensile Strength of Thin Glass,” Challenging Glass Conference, vol. 5, pp. 419 - 428, 2016.
[14] F. Oliveira Santos, C. Louter and J. Ramoa Correia, “Exploring Thin Glass Strength Test Methodologies,” Challenging Glass Conference Proceedings, vol. 6, pp. 713 - 724, 2018.
[15] M. Zaccaria and M. Overend, “Thermal Healing of Realistic Flaws in Glass,” Journal of Material in Civil Engineering, vol. 28, no. 2, 2016.
[16] K. C. Datsiou and M. Overend, “Artificial ageing of glass with sand abrasion,” Construction and Building Materials, vol. 142, pp. 536 - 551, 2017.